Keywords
|
Nitrous oxide, Endotracheal tubes, Diffusion |
Introduction
|
Nitrous oxide (N2O) is an inhalational anesthetic that is frequently used for general anesthesia. While N2O is not sufficient for maintaining general anesthesia if used alone, when used in combination with a volatile inhalation agent, N2O decreases the minimum alveolar concentration (MAC) of the volatile inhalational agent, thereby reducing the amount of the agent required during longer procedures [1]. However, N2O increases the intracuff pressure of the tracheal tube due to diffusion of N2O into the cuff during general anesthesia [2]. Intracuff pressure increases steadily and reaches a level high enough to impede the microcirculation in the tracheal mucosa within 1 h [3,4], which may cause damage to the tracheal tissue. This represents a major complication of N2O use. |
In anesthesia using N2O, it is thought that the N2O concentration in the tube cuff is close to the N2O concentration prescribed for the patient. Usually, at the end surgery, administration of N2O is stopped, and the patient is given 100% oxygen or air. Subsequently, the patient recovers from anesthesia and is moved to the intensive care unit. In such cases, the N2O that flowed into the cuff will be transferred to outside of the cuff, which may cause a decrease in cuff pressure. This decrease in cuff pressure may then trigger ventilator-associated pneumonia (VAP) [5]. However, few studies have examined the decrease in cuff pressure associated with N2O administration. Therefore, in this study, we examined the decrease in cuff pressure caused by the flow of N2O into the cuff. |
Materials and Methods
|
Tracheal tubes
|
The Portex Blue Line Profile, Soft-Seal Cuff (SPN) tube and Mallinckrodt Nasal RAE Tracheal Tube Cuffed, Murphy Eye (MRN) tube were used as test tracheal tubes in this study. The MRN tube was equipped with a normal polyvinyl cuff. Cuff filling volumes to achieve 30 cmH2O cuff pressure were 15.5 and 14 ml of air for SPN and MRN, respectively. |
Measurement procedures
|
Six gas preparations (air, oxygen, 70% N2O in oxygen, 50% N2O in oxygen, 30% N2O in oxygen, and TGM [a threegas mixture of 60% N2O in oxygen to air at a 4:3 ratio]) were analyzed (Table 1). SPN and MRN were inflated with the various gases under temperature conditions of 23°C. Initially, the cuff pressure was adjusted to 30 cmH2O by using cuff pressure manometers (VBM Cuff Controller Inflator from Smiths Medical) and was measured and recorded every 5 min for 60 min thereafter. These measurements were repeated five times for each group. All pressure measurements are presented in cmH2O. |
Statistical analysis |
Statistical analysis was performed on a personal computer by using SPSS 16.0 (SPSS Japan Inc., Japan) and Ekuseru-Toukei 2006 software (Social Survey Research Information Co. Ltd., Japan). All results are expressed as mean ± SD (SD). Homoscedasticity was tested using the Bartlett’s test. One-way repeated measures analysis of variance (ANOVA) was used for comparison of cuff pressures between the five gas groups (i.e., excluding the TGM group) followed by Dunnett’s test for multiple comparisons. In addition, Student’s t-tests were used for comparison of cuff pressures between the air and TGM groups. A probability of less than 0.05 was considered significant. Approximations were used to determine changing cuff pressures in each gas group. |
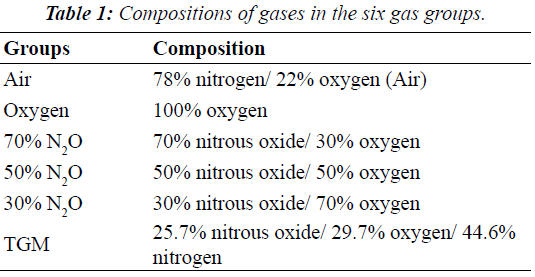 |
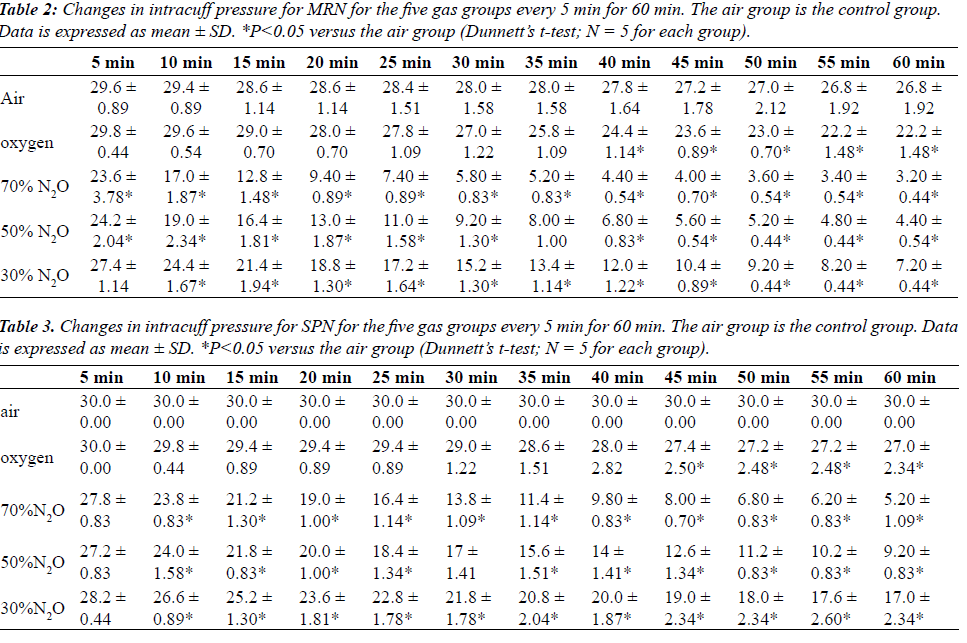 |
Results
|
Tables 2 and 3 shows mean changes in cuff pressures for MRN and SPN over time for the five gas groups (air, oxygen, 70% N2O, 50% N2O, and 50% N2O), and Figures 1 and 2 show deflation responses of MRN and SPN cuff pressures. In comparison with the air group, the oxygen group showed gradually decreasing cuff pressures, reaching significance at 40 min. Additionally, in the N2O groups, cuff pressures decreased more rapidly than in the oxygen group, with the rate of decrease being dependent on the concentration of N2O. In the 70% N2O group, cuff pressures changed more dramatically than those in the 50% and 30% N2O groups. Significant decreases were observed after only 10 or 20 min for MRN and SPN, respectively. By 60 min, cuff pressures in the 70% N2O group were 3.20 ± 0.44 and 5.20 ± 1.09 cmH2O for MRN and SPN, respectively, as compared with 26.8 ± 1.92 and 30.0 ± 0.0 cmH2O, respectively, for the control group. For the 70% N2O group, the mean durations required to reach 15 cmH2O were 12 and 27 min for MRN and SPN, respectively. Overall, significant relationships between sequential changes in cuff pressure and initial pressure were observed in all groups except the air group (control) for SPN. |
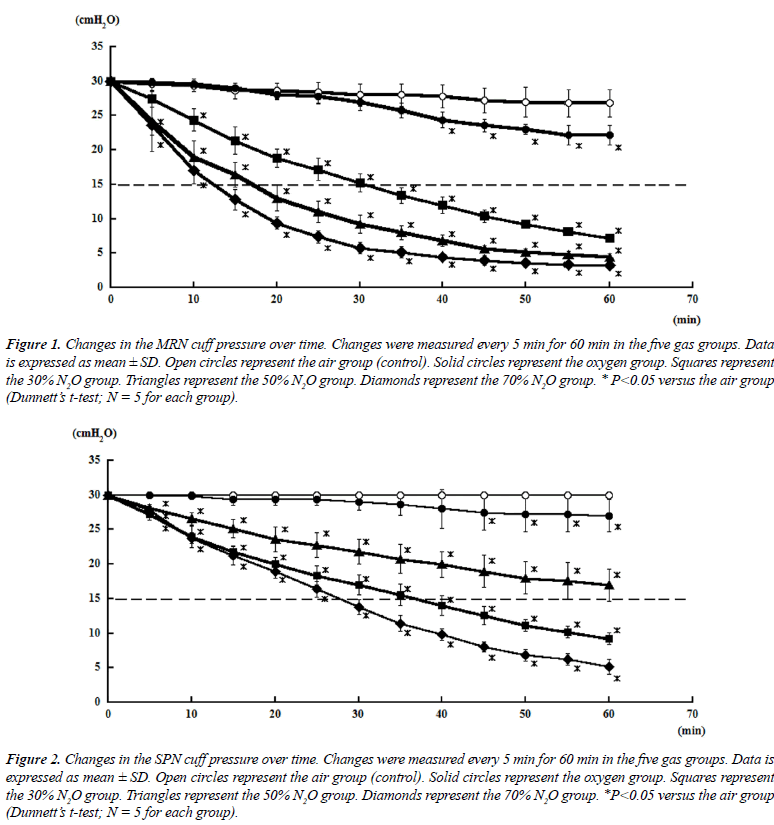 |
Cuff pressures in the SPN groups were consistently higher than those in the MRN groups throughout this study. However, SPN could not maintain the intra-cuff pressure sufficiently when inflated with N2O. |
Approximations of deflation in cuff pressure were determined for the five gas groups (i.e., air, oxygen, 70% N2O, 50% N2O, and 30% N2O; Tables 4 and 5). These approximations were assumed precise, as the coefficients of determination (R2 values) were 0.9749–0.9990, except for SPN in the air group. |
Tables 6 and 7 showed mean changes in cuff pressure over time for the TGM group. The composition of gases in this group was as follows: 25.7% N2O/29.7% oxygen/44.6% nitrogen (ratio of 60% N2O in oxygen to air = 4:3) [6]. For both MRN and SPN, the cuff pressures in the gas mixture group decreased significantly after 10 min compared with those in the air group; at 60 min, the cuff pressures reached 14.8 ± 2.77 and 17.4 ± 3.84 cmH2O for MRN and SPN, respectively (Figures 3 and 4). |
Discussion
|
In this study, we examined changes in cuff pressure following inflation of two types of tracheal tubes (MRN and SPN) with different gases. Our data demonstrated that inflation with N2O significantly decreased cuff pressures for both MRN and SPN; these data may help to predict cuff deflation in the clinical setting and provide insights into optimal use of N2O during anesthesia. |
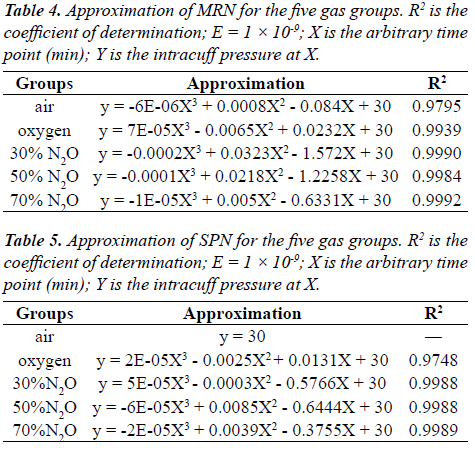 |
During general anesthesia, the pressure of the endotracheal tube cuff may need to be changed; in particular, the use of N2O during anesthesia results in increased cuff pressure due to the diffusion of N2O into the cuff [7], leading to damage to the tracheal epithelium and tracheal wall. However, N2O has major advantages for use during general anesthesia, facilitating rapid onset of anesthesia and improving recovery after anesthesia due to its low solubility in blood and adipose tissue. Furthermore, N2O decreases the MAC of other volatile inhalational agents when used in combination with N2O owing to the strong analgesic effects of N2O. Thus, these properties of N2O have led to the continued use of N2O in modern clinical practice. |
Various methods have been developed to limit the N2Odependent increase in cuff pressure. For example, several reports have described the use of a gas mixture containing the anesthetic gas with air or saline for inflation of the cuff [6,7]. Furthermore, specially designed tubes, such as the Brandt tracheal tube, which has a large pilot balloon that allows rediffusion of N2O [8,9], or the Hi-Lo Tracheal Tube with the Lanz System, which automatically maintains a low intracuff pressure, have been developed. Other devices that also control cuff pressure have been described [10]. However, these methods are not commonly used because of their increased cost and personal preference [11]. |
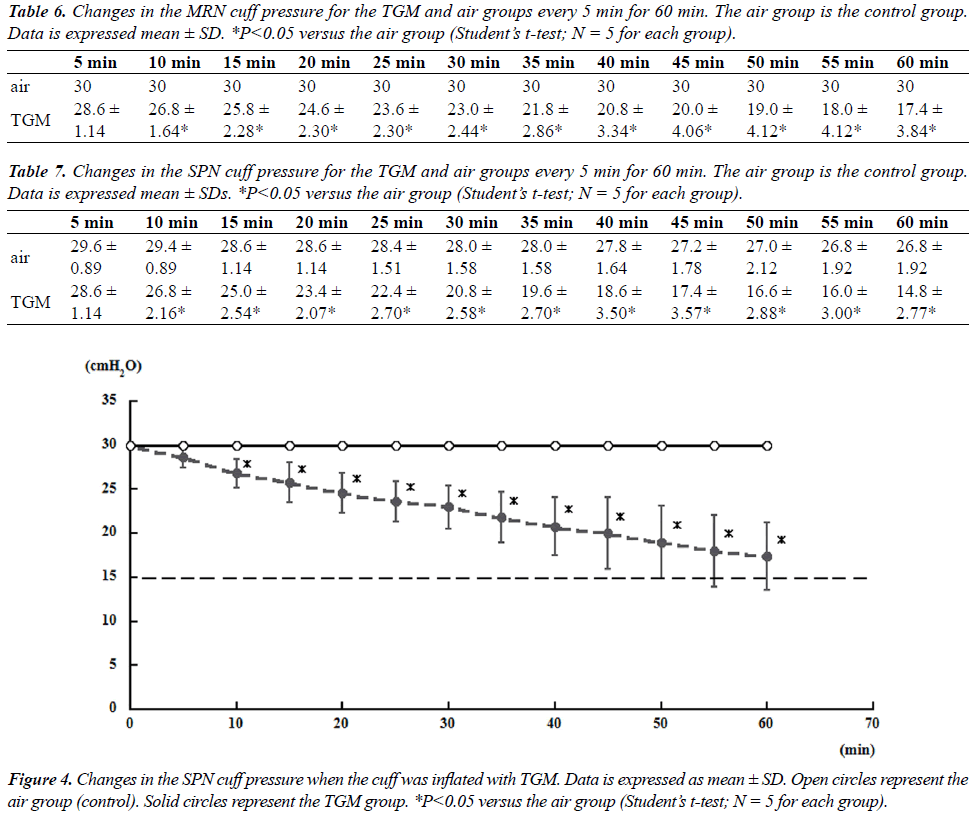 |
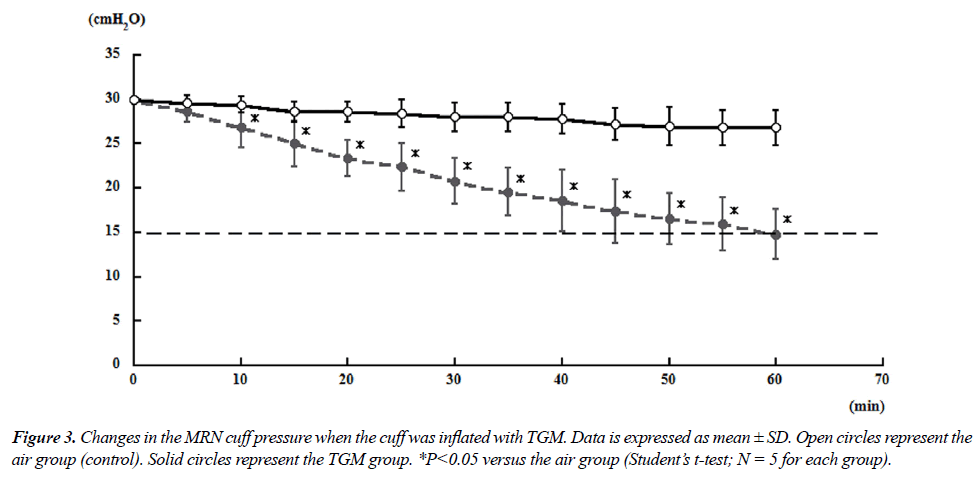 |
Modern tracheal tube cuffs are usually made of polyvinyl chloride (PVC), which is permeable to N2O. The MRN and SPN tubes used in this study were also made of PVC. Diffusion of N2O into a cuff is dependent on the permeability coefficient of the cuff wall and is inversely proportional to the thickness of the cuff wall [12]. The mean cuff thickness of both MRN and SPN was 0.06 mm. In SPN, however, a plasticizer is added to soften the PVC and to make the cuff less permeable to N2O [11]. In a previous study, SPN was shown to prevent the increase in intracuff pressure for 60 min after inflation of the cuff during N2O anesthesia, suggesting that SPN should be used to prevent high tracheal wall pressure [11,13]. On the other hand, a study by Karasawa et al. suggested that the main mechanism through which SPN prevents the increase in cuff pressure may be the high compliance of the thinner cuff, rather than the N2O gas-barrier properties [14]. In the current study, we found that cuff pressures were consistently higher when SPN was used than when MRN was used for all groups. Furthermore, higher concentrations of N2O led to more rapid changes in cuff pressure. Remarkably, no change in cuff pressure was observed at 60 min when SPN was used. However, both MRN and SPN exhibited significant decreases in cuff pressure after about 5 min when N2O was used to inflate the cuffs compared to that observed when air was used instead, suggesting that N2O diffused out of the cuff quickly. |
In a previous study, Manabe et al. showed that use of the Portex Profile Cuffed tube from Smiths Medical during general anesthesia led to a decrease in cuff pressure to 12 cmH2O at 60 min after inflation with inhalation gas (60% N2O/40% oxygen) [6], similar to the results of the 50% N2O in our study. Moreover, Manabe et al. reported that the cuff pressure of the mixture gas (inhalation anesthetic gas to air ratio = 4:3) exhibited almost no change during general anesthesia, measuring around 20 cmH2O at 60 min [6]. The composition of the mixture gas used by Manabe et al. was 25.7% N2O/29.7% oxygen/44.6% nitrogen, which is the same as that of the gas mixture used in our study. However, our results demonstrated a significant deflation of the cuff pressure when using MRN or SPN in the gas mixture group. Thus, from these results, we can conclude that MRN and SPN both allowed significant permeation of N2O in and out of the cuff. |
VAP is an infection related to mechanical ventilation [15] and has been reported to be the most prevalent nosocomial infection developing within 48 h of intubation in intensive care units [16]. The incidence of VAP is between 6% and 52% in intensive care units [17]. Among the factors predisposing patient to VAP, patient-related variables, including age and clinical condition, as well as treatment and care practices, such as enteral feeding and antacid prophylaxis, and factors related to infection control have been reported [18,19]. Moreover, decreased tube cuff pressure and cuff-stricture are associated with the etiology of VAP [5]. Thus, management of cuff pressure during general anesthesia and ICU management is important for prevention of VAP. |
Our study used tracheal tube cuffs exposed to the atmosphere; this study model may be beneficial for the study of decreased cuff pressure. However, this study is only a pilot study on VAP prevention, and further studies are required to determine whether these results translate to intubated patients during general anesthesia or management in the intensive care unit. Currently in our laboratory, we are involved in a study of decreased cuff pressure during artificial ventilation. |
In conclusion, SPN showed low permeability to N2O. However, the cuff pressure decreased according to the concentration of N2O. Consequently, we propose that anesthesiologists must always consider cuff pressure when using N2O. Indeed, a rapid decrease in cuff pressure may occur after administration of N2O, which could increase the risk of VAP. Accordingly, it is essential to continually monitor cuff pressure during recovery from anesthesia and while moving the patient after surgery. |
Acknowledgements
|
This study was supported by Kyushu University. A part of this study was presented at Euroanaesthesia, Barcelona on 3 June 2013. The authors thank Kunio Suwa, MD, PhD for careful reading and editing of the manuscript. The authors would also like to thank the editors at Editage for their assistance in editing and proofreading the manuscript. |
Conflicts of Interest
|
None. |
Funding Sources
|
None. |
|
References
- 1. Eschertzhuber S, Salgo B, Schmitz A, Roth W, Frotzler A, Keller CH, Gerber AC, Weiss M. Cuffed endotracheal tubes in children reduce sevoflurane and medical gas consumption and related costs. Acta Anaesthesiol Scand 2010; 54: 855- 858.
- 2. Stanley TH, Kawamura R, Graves C. Effects of nitrous oxide on volume and pressure of endotracheal tube cuffs. Anesthesiology 1974; 41: 256-262.
- 3. Raeder JC, Borchgrevink PC, Sellevold OM. Tracheal tube cuff pressures. The effect of different gas mixtures. Anaesthesia 1985; 40: 444-447.
- 4. Fujiwara M, Mizoguti H, Kawaura I, Takakuwa R, Morinaga N, Yanagawa T, Chiba T, Odashiro M. A new endotracheal tube with a cuff impervious to nitrous oxide: constancy of cuff pressure and volume. Anesth Analg 1995; 81: 1084-
- 1086. 5. Hwang YJ, Han HS, Park HS, Park JS, Park S, Oh HS, Kim JH. Interrupting gel layer between Double cuffs prevents fluid leakage past tracheal tube cuffs. Br J Anaesth 2013; 111: 496-504.
- 6. Manabe M, Ookawa I, Tanaka Y, Kunazawa T. Tracheal tube cuff pressure. Anaesthesia 1987; 1: 173-177.
- 7. Revenas B, Lindholm CE. Pressure and volume changes in tracheal tube cuffs during anaesthesia. Acta Anaesthesiol Scand 1976; 20: 321-326.
- 8. Brandt L, Miller-Spath R, Moussa RG. Reduction in nitrous oxide induced endotracheal tube cuff pressure during anaesthesia with the ?Rediffusion System’. 2nd Ed. Mallinckrodt Sci Ed Booklet, Germany, Mallinckrodt, Hennet/Sie 1990.
- 9. Karasawa F, Okuda T, Mori T, Oshima T. Maintenance of stable cuff pressure in the BrandtTM tracheal tube during anaesthesia with nitrous oxide. Br J Anaesth 2002; 89: 271- 276.
- 10. Payne KA, Miller DM. The Miller tracheal tube cuff pressure control valve. Anaesthesia 1993; 48: 324-327.
- 11. Al-Shaikh B, Jones M, Baldwin F. Evaluation of pressure changes in a new design tracheal tube cuff, the Portex Soft Seal, during nitrous oxide anaesthesia. Br J Anaesth 1999; 83: 805-806.
- 12. Chandler M. Pressure changes in tracheal tube cuffs. Anaesthesia 1986; 41: 287-293.
- 13. Umezono Y, Fujita A, Toi T, Sakio H. Usefulness of tracheal tube with N2O gas-barrier cuff. Masui 1999; 48: 1250-1252.
- 14. Karasawa F, Mori T, Okuda T, Satoh T. Profile soft-seal cuff, a new endotracheal tube, effectively inhibits an increase in the cuff pressure through high compliance rather than low diffusion of nitrous oxide. Anesth Analg 2001; 92: 140-144.
- 15. ?ahino?lu H, Dilek A. Mekanik ventilasyon komplikasyonlar?: In: ?ahino?lu H eds. Yo?un Bak?m Komplikasyonlar?, Nobel T?p Kitabevleri, Turkish 2008; pp 29-37.
- 16. Augustyn B. Ventilator-associated pneumonia – risk factors and prevention. Crit Care Nurse 2007; 27: 32-36.
- 17. Davis KA. Ventilator-associated pneumonia: a review. J Intensive Care Med 2006; 21: 211-226.
- 18. Gündo?du C. Ventilatör ba??ml? pnömoniler: In: Numano?lu N, Willke (Topçu) A eds. Güncel Bilgiler I????nda Pnömoniler, Bilimsel T?p Yay?nevi, Turkish 2000; pp 357-362.
- 19. Kahraman BB, Ozdemir L. The impact of abdominal massage administered to intubated and enterally fed patients on the development of ventilator-associated pneumonia: a randomized controlled study. Int J Nursing Studies 2015; 52: 519-524.
|