Background and aim: In dental treatments, use of antibiotic carriers can decrease microorganisms more efficiently. In this study, gentamicin (GEN) was loaded onto poly lactic-co-glycolic acid (PLGA) copolymers and PLGA-nano hydroxyapatite (nHA) composite microspheres for a controlled release. The amount of drug release was measured for 20 days. Materials and methods: In this in-vitro study, microspheres were prepared using the water/oil/ water technique and different concentration of GEN loaded onto PLGA copolymers and PLGA- nHA composite microspheres. Loaded microspheres were evaluated morphologically using scanning electron microscopy (SEM). The rate of drug release from the composite microspheres was measured in phosphate buffered saline (PBS) medium for a period of 20 days using UV spectrophotometry (330 nm). Data were analyzed using ANOVA. Also, a microbial culture was carried out of microspheres with the least amount of drug in the first and last day of drug release assessment. Results: SEM images showed that the microspheres had a smooth surface and the pattern of drug release from the PLGA copolymers and PLGA-nHA composite microspheres loaded with GEN was different in each group at different time points, but this difference only in the PLGA+0.02 GEN group was significant. There was a significant difference between this group and other groups in the amount of released drug at day 6. Also, the results of microbial culture of the group with 0.02 GEN showed the antibacterial effect of these microspheres in the last day of experiment. Conclusion: The release profiles observed in this study and the well-established biocompatibility of PLGA indicate that the composite microspheres used in this study are suitable for infection control purposes in dentistry.
Keywords |
Gentamicin; Drug delivery; Composite microspheres; Nano-Hydroxyapatite; Poly lactic-co-glycolic acid |
Introduction |
Systemic antibiotic therapy has several complications [1]
and exerts some adverse effects on several body organs
mainly the kidneys, ears and liver. Therefore, finding a
method for targeted local delivery of antibiotics can greatly
decrease the undesired complications associated with
their systemic use. Moreover, systemic use of antibiotics
has small efficacy for dental infections unless they are administered in high doses [2]. Thus, local delivery of
antibiotics to the desired site can enable greater efficacy
at a significantly lower dose for treatment of dental
infections. Advances in tissue engineering have paved the
way for the use of different antibiotics loaded onto polymer
microsphere carriers for dental purposes. |
Direct pulp capping is a common treatment in case of
traumatic or mechanical pulp exposure. In this treatment, the exposed pulp is covered with a specific material
allowing the pulp to form reparative dentin at the exposure
site. Formation of a dentin barrier is often followed by
dental pulp recess while the tooth remains vital. Calcium
hydroxide (CH) is the standard pulp capping material for
vital pulp treatment [3]. It possesses antibacterial effects
and can induce the formation of a microscopic calcified
barrier. However, this barrier cannot reinstate a permanent
seal and can lead to bacterial leakage. Also, based on the
literature, the success rate of vital pulp therapy is not
acceptably [4,5] high in the primary teeth [6]. |
Nano-hydroxyapatite is a bioactive, biocompatible
compound highly accepted for use in dental and
medical science and has antibacterial effects [7]. Nanohydroxyapatite
is preferred to HA due to its greater surface
area and higher solubility [8]. The biological properties of
nHA are superior to those of HA. Combining nHA with
biocompatible polymers prevents nano powder wash out
[9,10]. |
The efficacy of amoxicillin, vancomycin, erythromycin and
doxycycline against Enterococcus faecalis has been well
investigated [11-13]. Use of systemic and local antibiotic
therapy has long been accepted as a standard protocol in
medicine and dentistry. In local use of antibiotics, their
systemic side effects are prevented and higher therapeutic
concentrations can be achieved [14]. Enterococcus faecalis
is an anaerobic Gram-positive bacterium and a member
of normal flora of the mouth. It is often found in small
amounts in unprepared root canals. However, its exact role
in the success of root canal treatment has yet to be clearly
identified. It is the most commonly isolated bacterium
from the root canal system. This microorganism can cause
a treatment-resistant infection due to its ability to invade
dentinal tubules, resistance against different ecological
conditions of the canal and adaptation to unfavorable
intracanal conditions [15]. |
Manufacturing dental cements, which contain
biodegradable polymer microspheres for controlled release
of antibiotics without affecting the mechanical properties
of cements, is increasing [16,17]. In this method, drugs are
loaded on the surface and inside synthetic microspheres
of variable sizes. These microspheres are biocompatible
and biodegradable and can be easily converted into threedimensional
matrixes with diverse structures. |
Biodegradable synthetic polymers include linear aliphatic
polyesters, polyanhydrides and poly (ortho esters); among
which, linear aliphatic polyesters namely polylactic acid,
polyglycolic acid and their copolymers have extensive
applications in local drug delivery systems [18]. Biological
products such as glycolic and lactic acid monomeric units
are naturally produced and then biodegraded in metabolic
pathways of the human body. Their difference is in their
structure and rate of degradability. Polyglycolic acid is
degraded more rapidly than polylactic acid; however, the
PLGA (50:50) has suitable properties for drug delivery. On the other hand, the behavior and the biodegradability
of PLGA are controllable and these characteristics are the
main advantages of this polymer and the reason for its vast
application in medical and dental fields [19-21]. |
Use of biodegradable polymers as microparticles loaded
with medications is a suitable alternative to some complex
medical and dental procedures. These microspheres
are mostly made of PLGA and are used for in-vitro proliferation of cells and are injected into the injured site
for the repair of cartilaginous tissue [22-24]. |
Several pharmacological and orthopedic studies have
investigated the production of PLGA microspheres and
assessed the release of loaded antibiotics. In restorative
dentistry, pulp-capping agents are used with the aim
of eliminating microorganisms from the pulp chamber.
Thus, it is particularly important to use antibiotic-loaded
materials to minimize the microbial load as much as
possible [25]. |
Our previous studies have been revealed that PLGA 50:50
microspheres are suitable carrier for loading of gentamicin
antibiotic [26]. This study sought to assess the rate of
release of gentamicin loaded onto PLGA and PLGA-nHA
microspheres. In this study, we investigate the role of nano
HA in composite microsphere and compared releasing
profile in different concentration of gentamicin sulfate
solution loaded in PLGA and composite PLGA/nano HA
microspheres. |
Materials and Methods |
Materials |
Poly (lactic-co-glycolic acid) and PBS were purchased
from Sigma Company (Sigma, Missouri, United States).
Gentamicin (GEN) was purchased from Sina Darou
Company (Sina Darou, Karaj, Iran). Polyvinyl alcohol
(PVA, 87-89% hydrolyzed, mol. wt. 31,000-50,000 g/
mol) was purchased (Sigma, United States). Chloroform
was purchased from Merck Company (Merck, Darmstadt,
Germany). Nano-hydroxyapatite was prepared in the
Materials and Energy Research Center. |
Methods |
This in-vitro experimental study evaluated the release
of different concentrations of GEN from PLGA and
PLGA-nHA microspheres. Non-probability convenience
sampling was carried out. |
Microsphere preparation |
Water-in-oil-in-water (W/O/W) double emulsion/solvent
evaporation technique was used for the preparation
of microspheres. Briefly, 1 ml of 15% (w/v) PLGA in
chloroform was added to 2 ml of internal water phase
[PVA 2% (w/v)] containing different concentrations
of GEN [0.1, 0.025 and 0.05% (w/v)] and different
concentrations of nHA [0 and 25% (w/w) of polymer]. The
mixture was homogenized for one minute at 10,000 rpm
to achieve water/oil (W/O) emulsion. After that, the W/O emulsion was poured into 30 ml of the second aqueous
phase containing 0.2% (w/v) PVA (Sigma, (PVA, 87-89%
hydrolyzed, mol. wt. 31,000-50,000 g/mol) and mixed
with stirrer (Heidolph, Schwa Bach, Germany) for two
hours to prepare W/O/W emulsion. The microspheres were
then collected and washed three times with distilled water
by centrifugation (11000 rpm, 10 min) after final washing;
the samples were collected and frozen at -15°C. Finally,
the samples were lyophilized by freeze-dryer (Christ
ALPHA 1-2 LD PLUS, SY4 5NU, UK) for 48 hours at
-15°C. Table 1 summarizes the mean concentration of
drug released in different groups at different time points. |
Microsphere characterization |
Scanning Electron Microscopy (SEM): The size,
morphology and microstructure of microspheres
were analyzed using SEM (VEGA TESCAN-LMU,
Kohoutovice, Czech Republic). Samples were fixed on an
aluminum plate and gold coated prior to examination. |
Determination of gentamicin encapsulation efficiency: To determine the loading efficiency of GEN, 15 mg of the
drug-containing microspheres were poured into vials and
5% w/v SDS in 0.1 molar NaOH was added. The solution
was mixed with stirrer in order for the microspheres to
disintegrate and allow complete release of medication.
After 12 hours, the mixture was centrifuged at 8000 rpm
for 5 minutes for complete isolation of the remaining
particles. The supernatant was collected. The amount of
drug extracted from the microspheres was measured and
compared with the baseline drug value before loading.
The drug encapsulation efficiency was calculated using
the following equation: |
Encapsulation Efficiency (EE%) = [drug (encapsulate)/
drug (total)] ×100 |
All the tests were performed in triplicate, and the results
were reported as mean ± standard deviation (SD). Table 2. |
In-vitro drug release study: For in-vitro drug release
study, 14 mg of different samples were suspended in 3 ml
of PBS (pH: 7.4). The plates were then placed in a shaker
incubator (Behdad, Tehran, Iran) at 37°C rotating at 100
rpm. At pre-determined times; the PBS was completely
extracted and replaced with 3 ml of fresh PBS. The amount
of GEN released into the PBS was quantified by UV-V at
330 nm and compared with a standard calibration curve.
All drug release studies were carried out three times. |
Bacterial culture: A 24-hour Staphylococcus aureus
culture was prepared in brain heart infusion (BHI) agar
at 37°C. Twenty-four hour cultured single colonies were
removed and inoculated in saline solution to prepare
a 0.5 McFarland standard bacterial suspension. The
suspension was streak cultured on BHI agar plate. Using
a Pasteur pipette, 100 γ of the control, PLGA+0.02 GEN
and PLGA/nHA+0.02 GEN solutions were added to the
wells. The plates were then incubated at 37°C. After 24
hours, the plates were evaluated for the formation of
growth inhibition zones. Next, another 24-hour culture of
Staphylococcus aureus was grown and 0.05 McFarland
standard microbial suspensions was prepared. Then, 100
γ of the broth medium was added to each well of the 96-
well plate. Then, 100 γ of the highest concentration was
added to the first well containing broth medium. Next,
100 γ was removed from the first well and added to the
second one and so on; the final 100 γ was removed from
the last well and discarded. Serial dilution was carried
out as such. Next, 100 γ of the 0.5 McFarland standard
microbial suspension was added to each well and then the
plate was incubated at 37°C in an incubator (Memmert,
Schwa Bach, Germany). After 24 hours, the turbidity was
assessed to determine minimum inhibitory concentration.
In the two experimental groups of PLGA+0.02GEN and
PLGA/nHA+0.02GEN that contained the least amount
of GEN, microbial cultures were prepared at the first and
last day. The growth inhibition zone of S. aureus was evaluated. |
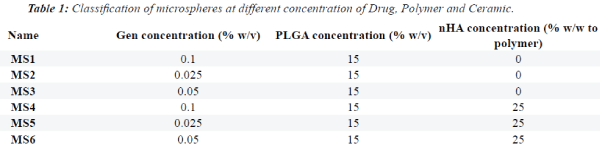 |
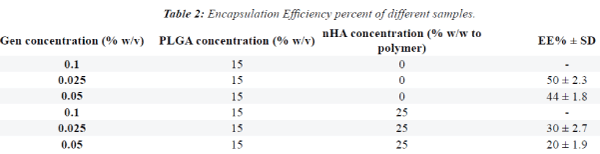 |
Statistical Analysis |
Data were analyzed using SPSS 18. The mean, SD,
minimum and maximum concentration of the released
drug in different groups at different time points were
calculated. The data were analyzed using one-way and
two-way ANOVA. Type 1 error was considered as 0.05
and P<0.05 was considered statistically significant. |
Results |
Figures 1 and 2 show SEM images. GEN-loaded PLGA
microspheres had a smooth surface without any pores
or breakage. The SEM images showed that the size of
microspheres containing GEN was approximately 30
microns (values represent mean ± SD). |
The mean concentration of the released drug in different
samples was plotted as a function of time. Figures 3 and 4
shows the release of GEN from microspheres over 20 days.
These figures showed a constant and controlled release
profile. Although the pattern of release was different at
different time points, overall, the pattern of release was
not significantly different among groups and the effects of
drug concentration on the amount of released drug were
not significant (P=0.26). |
One-way ANOVA demonstrated that no significant
difference existed in the concentration of released drug at different time points except for day 6, which revealed a
significant difference in the concentration of the released
drug (P<0.003). On day 6, burst release occurred in the
PLGA+0.02 GEN; which was significantly different from
the pattern of release in other groups. Drug release in
different groups and at different time points is demonstrated
in Figure 5. |
Since the plasma concentration of drug after systemic
use was 24 μg/ml, the mean concentration at day one
in the 0.02 GEN group was around 10-7 g/ml; which
was significantly lower than 24 μg/ml. Thus, no growth
inhibition zone was observed in the culture medium. On
the last day, this rate reached 10-5 g/ml, which was within
the effective dose and a growth inhibition zone measuring
16-18 mm was observed. It should be noted that these
values are calculated based on the mean release of drug
in the same day. If the effective dosage under in-vivo
conditions is considered, drug shows a cumulative effect
and the drug value from day 6 on will be in the effective
therapeutic range. In PLGA-nHA+0.02 GEN group, the
mean release on day one was in the range of 10-10 and from
day 6 on, it reached the effective dose of 10-6 g/ml. Thus,
the effective dose is released from day 6 on due to the
cumulative effect. In PLGA-nHA+ 0.05 GEN, the release
was slow until day 8 but from day 10 on, the speed of
release accelerated and reached the effective dosage for
inhibiting bacterial growth (about 10-6). |
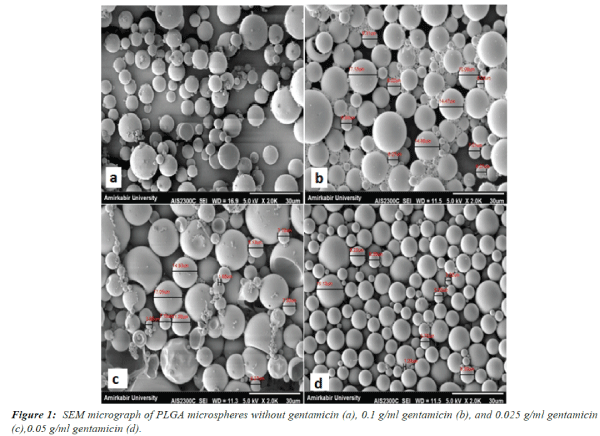 |
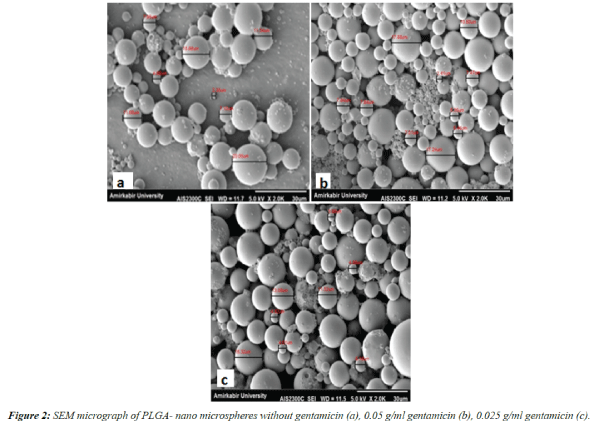 |
Discussion |
The current study assessed the GEN release profile when
different combinations of drug and PLGA and nHA were
used. The results showed that HA had no negative effect
on the drug release rate and therefore, it can be used to
help pulp regeneration. Moreover, powders containing
polymer and antibiotic microspheres may be mixed with
mineral trioxide aggregate for efficient use in direct pulp
capping and at the site of pulp exposure. Based on the
results of the current study, daily drug release profile was
variable in different groups but the overall release profile
of the groups was similar. However, the release rate was
the highest in PLGA+0.02GEN group on day 6, which
may be due to the homogenous and uniform distribution
of microspheres in this group compared to others. The
difference in release rate was not significant on the final
day of experiment among groups. |
It is believed that nHA has special properties due to its
small particle size and huge specific surface area. A
significant increase in protein adsorption and osteoblast
adhesion to nano-sized ceramic materials was reported by
Webster et al [27,28]. SEM images in our study showed
that microspheres were 30 μm and had a smooth surafce.
This result is in agreement with the findings of Sivakumar
et al., (2002) who created 16 μm microspheres [29].
However, the difference was in the surface characteristics
of microspheres because in our study micropsheres had a smooth surface but in their study microspheres had a
porous surafce. The more porous the surface, the better
the results. |
Increasing the volume of secondary aqueous phase
resulted in higher encapsulation efficiency. As the size
of microspheres increases their degradation rate can be
more easily controlled; but in our study, the smaller size of
microspheres resulted in lower encapsulation efficiency.
Also, in our study, different groups had the same drug
release profile, beacause the drug was loaded onto
homogenous microspheres with almost equal sizes. |
The group containing 0.02 GEN, had more microspheres
with smaller size and more uniform distribution. |
Smaller microspheres have a biphasic release profile
with a quick second phase. The three-phasic release
profile mostly occurs in larger microspheres; which is the
result of heterogeneous destruction. If the particles are
of various sizes, the release profile will be single phase.
In three-phasic release profiles, the first phase usually
includes an initial burst causing the release of nonencapsulated
drug molecules or those over the surface
of microspheres. The release is followed by small water
crack formation and primary degradation of microspheres
may also be responsible for the initial burst [30]. However,
we did not observe this on SEM images in our study.
The second phase includes slow release of the drug. At
the same time, polymer destruction continues along with water absorption. The third phase is faster and occurs as
the result of polymer mass loss due to the destruction of
PLGA. The third phase is sometimes called the second
burst [31]. |
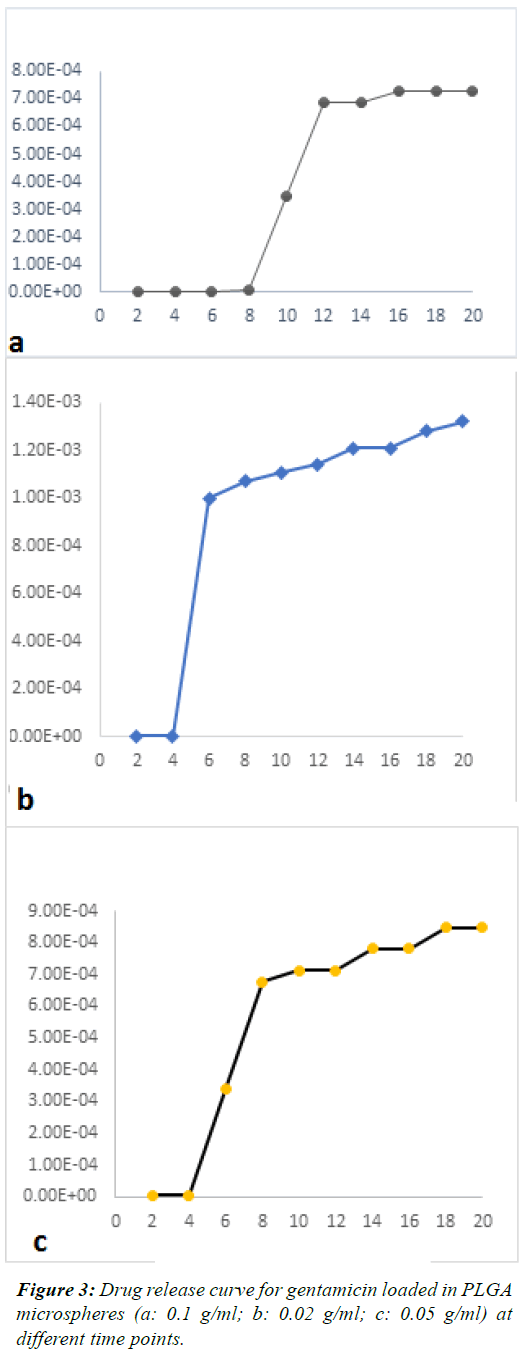 |
Vitro et al. (2007) [32] showed that using ultrasonic
method for prepration of microspheres not only increased encapsulation effiecncy in size of 20-40 μm
but also improved the encapsulation effiecncy of GEN
to almost 100%; but in our study the ultrsound method
was not employed and thus, the microsphere size and
the encapsulation effiecncy of GEN were lower. In
future studies, ultrasonic method can be used to improve
encapsulation effiecncy. |
Sousa et al. loaded PLGA microspheres with amoxicillin
[33]. In their study, microparticles measuring 5-38 μm
were prepared using a spray-drying technique and different
drug-release patterns were observed. Drug composition
played a significant role in the controlled release profile.
The antibacterial activity of amoxicillin continued even
after its encapsulation. As demonstrated by antibiogram
results, amoxicillin had antimicrobial effects for 6 hours.
Spongy particles in contact with water enhance complete
elimination of the formulation from the simulated root
canal system. However, this study, similar to the previously
discussed ones, was conducted under invitro conditions;
which has significant differences with the clinical setting. |
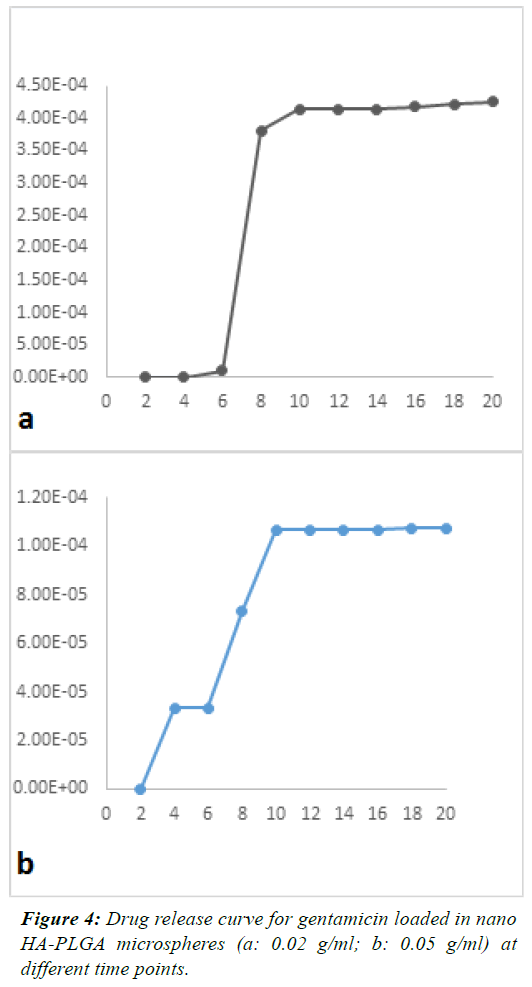 |
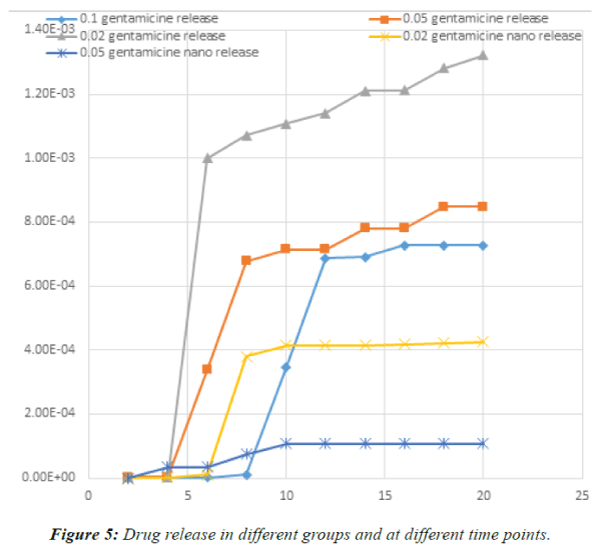 |
In addition, the results of the bacterial culture showed that
GEN concentration was 24 μg/ml after systemic use. The
mean GEN concentration in PLGA+0.02 GEN sample
was 7-10 μg/ml on the first day and the inhibition zone
was not observed. On the last day, GEN concentration in
this sample reached 5-10 μg/ml, which was within the
effective range and the inhibition zone was calculated at
the range of 16-18 mm. The GEN release from PLGAnHA+
0.02 GEN sample was 10 μg/ml on the first day and
it was within the effective range on the eighth day (10-6 μg/ml). Until the sixth day, the PLGA-nHA+0.05 GEN
sample showed prolonged release. Thus, the samples
containing nHA have more controlled release profile than
pure PLGA microspheres. |
PLGA +0.02% GEN showed the highest rate of delivery
and burst release on the sixth day, which had significant
differences with other groups. This finding can be explained
by the fact that less amount of loaded drug causes faster
release in camparison to loading 0.05% and 0.1% GEN.
According to a study by Imbuluzqueta et al., in 2011 [34],
the higher the amount of the drug, the more the interaction
with the microspheres and the slower the release profile.
In the study of Imbuluzqueta et al, the efficiency of nanoparticles
loaded with GEN was approximately 100% and
the sustained release was achieved for up to 70 days. This
difference is due to the GEN coupling with the anionic
AOT salt [(salt-2) BISsulfosuccinate sodium (ethylhexyl)]
and the formation of GS-AOT hydrophobic complex. |
In our study, double emulsion method was used for
drug preparation. However, Prior et al. (2000) [35]
prepared GEN particles using the spray drying method.
Although they showed continuous burst of drug, it caused
agglumeration of particles compromising drug release. |
Our study showed that by increasing the concentration of
GEN, drug loading into the microspheres decerased; this
finding is in accord with the results of a previous study by
Blanco-Prieto et al., in 2002 [36]. |
Schneiders et al., in 2006 (Schnieders et al., 2006)
evaluated the characteristics of composite microspheres
(calcium phosphate PLGA) and observed no reduction in
mechanical properties of these cements. Drug loading was
also successful. Similarly, nHA and PLGA were mixed;
this mixture had no adverse effect on the properties of the
two materials, and addition of nHA increased the molecular
weight of the composite and better controlled the drug
release. It is quite clear that microspheres containing nHA
have a slow release profile. |
Studies have shown that different formulations, the speed
of mixing, chemical composition, surface activator,
viscosity of the polymer solution and the volumetric
ratio of aqueous phase to organic phase can affect the
characteristics and properties of polymer microspheres.
Our results showed that double emulsion method was
suitable for preparation of PLGA microspheres containing
GEN. Also, the release profile showed that GEN molecules
were released from the PLGA microspheres via a controlled
mechanism by penetration and destruction of polymer in 3
phases: 1. Controlled release via penetration mechanism,
2. Penetration mechanism and simultaneous degradation
causing initial burst, and 3. Delayed, controlled and slow
drug release. PLGA microspheres have slow, continuous
release profile; which is the reason for the popularity of
this system. This study appears that PLGA+GEN and
PLGA-nHA+GEN may also be mixed with MTA and
placed over the exposed site to increase the success rate
of pulp capping. Habruken et al, in 2010 confirmed these findings as well [37]. |
Future studies are required to assess drug release in
the clinical setting. Also, the effect of adding more
hydrophilic surfactants on the drug release profile must
be evaluated. The release profile of amoxicillin and other
antibiotics must be evaluated as well. Assessment of the
physical properties of cements containing drug-loaded
microspheres would also be an interesting research topic. |
Conclusion |
In loading GEN onto PLGA and PLGA-nHA microspheres,
no difference was noted in the daily release pattern of drug
in groups with different concentrations of drug. On day
6, burst release of drug occurred in the PLGA+0.02%
GEN group, which was significantly different from other
groups. GEN can be loaded onto PLGA-nHA composite
microspheres for use in restorative treatments like direct
pulp capping. |
References |
- Alexandra K Marr, WJGAREH. Antibacterial peptides for therapeutic use: obstacles and realistic outlook. Current Opinion in Pharmacology 2006; 6: 468-472.
- Kapoor A, Malhotra R, Grover V, Grover D. Systemic antibiotic therapy in periodontics. Dental research journal 2012: 9: 505.
- Dammaschke T, Camp JH, Bogen G. 4 MTA in Vital Pulp Therapy. Mineral Trioxide Aggregate: Properties and Clinical Applications 2014; 71.
- Pace R, Giuliani V, Pagavino G. Mineral trioxide aggregate as repair material for furcal perforation: case series. Journal of endodontics 2008; 34: 1130-1133.
- Farsi N, Alamoudi N, Balto K, AL Mushayt A. Clinical assessment of mineral trioxide aggregate (MTA) as direct pulp capping in young permanent teeth. Journal of Clinical Pediatric Dentistry 2007; 31: 72-76.
- Haghgoo R, Naderi NJ. Comparison of calcium hydroxide and bioactive glass after direct pulp capping in primary teeth. Journal of Dentistry of Tehran University of Medical Sciences 2007; 4: 155-159.
- Saravanan S, Nethala S, Pattnaik S, Tripathi A, Moorthi A, Selvamurugan N. Preparation, characterization and antimicrobial activity of a bio-composite scaffold containing chitosan/nano-hydroxyapatite/nano-silver for bone tissue engineering. International journal of biological macromolecules 2011; 49:188-193.
- Kalita SJ, Bhardwaj A, Bhatt HA. Nanocrystalline calcium phosphate ceramics in biomedical engineering. Materials Science and Engineering 2007; 27: 441-449.
- Sawicki L, Pameijer CH, Emerich K, Adamowicz-Klepalska B. Histological evaluation of mineral trioxide aggregate and calcium hydroxide in direct pulp capping of human immature permanent teeth. American journal of dentistry 2008; 21: 262-266.
- NG F, Messer LB. Mineral trioxide aggregate as a pulpotomy medicament: an evidence-based assessment. European Archives of Paediatric Dentistry 2008; 9: 58-73.
- Dahlén G, Samuelsson W, Molander A, Reit C. Identification and antimicrobial susceptibility of enterococci isolated from the root canal. Oral microbiology and Immunology 2000; 15: 309-312.
- Pinheiro E, Gomes B, Ferraz C, Teixeira F, Zaia A, SouzaFilho F. Evaluation of root canal microorganisms isolated from teeth with endodontic failure and their antimicrobial susceptibility. Oral microbiology and Immunology 2003; 18: 100-103.
- PinheiroE, GomesB, DruckerD, ZaiaA, FerrazC, Souza‐FilhoF.Antimicrobial susceptibility of Enterococcus faecalis isolated from canals of root filled teeth with periapical lesions. International endodontic journal2004; 37: 756-763.
- Goodson J. Pharmacokinetic principles controlling efficacy of oral-therapy. Journal of Dental Research 1989; 68: 1625-1632.
- Kayaoglu G, Ørstavik D. Virulence factors of Enterococcus faecalis: relationship to endodontic disease. Critical Reviews in Oral Biology & Medicine 2004; 15: 308-320.
- Schnieders J, Gbureck U, Thull R, Kissel T. Controlled release of gentamicin from calcium phosphate—poly (lactic acid-co-glycolic acid) composite bone cement. Biomaterials 2006; 27: 4239-4249.
- SimonCG, KhatriCA, WightSA, WangFW.Preliminary report on the biocompatibility of a moldable, resorbable, composite bone graft consisting of calcium phosphate cement and poly (lactide‐co‐glycolide) microspheres. Journal of orthopaedic research 2002; 20: 473-482.
- Pillai O, Panchagnula R. Polymers in drug delivery. Current opinion in chemical biology 2001; 5: 447-451.
- Gunatillake PA, Adhikari R. Biodegradable synthetic polymers for tissue engineering. Eur Cell Mater 2003; 5: 1-16.
- ShiG, CaiQ, WangC, LuN, WangS, BeiJ.Fabrication and biocompatibility of cell scaffolds of poly (L‐lactic acid) and poly (L‐lactic‐co‐glycolic acid). Polymers for advanced technologies2002; 13: 227-232.
- Lavik E, Langer R. Tissue engineering: current state and perspectives. Applied microbiology and biotechnology 2004; 65: 1-8.
- Mercier NR, Costantino HR, Tracy MA Bonassar LJ. A novel injectable approach for cartilage formation in vivo using PLG microspheres. Annals of biomedical engineering 2004; 32: 418-429.
- Kang, SW, Jeon O, Kim BS. Poly (lactic-co-glycolic acid) microspheres as an injectable scaffold for cartilage tissue engineering. Tissue engineering 2005; 11: 438-447.
- Thissen H, Chang KY, Tebb T, Tsai WB, Glattaue RV, Ramshaw J, Werkmeister J. Synthetic biodegradable microparticles for articular cartilage tissue engineering. Journal of Biomedical Materials Research Part A 2006; 77: 590-598.
- Campoccia D, Montanaro L, Speziale P, Arciola CR. Antibiotic-loaded biomaterials and the risks for the spread of antibiotic resistance following their prophylactic and therapeutic clinical use. Biomaterials 2010; 31: 6363-6377.
- H. Nojehdehian ME, Z JaberI Ansari. Loading of Gentamicin Sulfate into Poly (Lactic-Co-Glycolic Acid) Biodegradable Microspheres. Journal of Dental School 2015; 33: 145-151.
- Webster TJ, Ergun C, Doremus RH, Siegel RW, Bizios R. Specific proteins mediate enhanced osteoblast adhesion on nanophase ceramics. Journal of biomedical materials research 2000; 51: 475-483.
- Dhivya S, Saravanan S, Sastry T, Selvamurugan N. Nanohydroxyapatite-reinforced chitosan composite hydrogel for bone tissue repair in vitro and in vivo. Journal of nanobiotechnology 2015; 13: 40.
- Sivakumar M, Rao KP.Preparation, characterization and in vitro release of gentamicin from coralline hydroxyapatite–gelatin composite microspheres. Biomaterials 2002; 23: 3175-3181.
- Fredenberg S, Wahlgren M, Reslow M, Axelsson A. The mechanisms of drug release in poly (lactic-co-glycolic acid)-based drug delivery systems—a review. International journal of pharmaceutics 2011; 415: 34-52.
- Jain RA. The manufacturing techniques of various drug loaded biodegradable poly (lactide-co-glycolide)(PLGA) devices. Biomaterials 2000; 21: 2475-2490.
- Virto MR, Elorza B, Torrado S, Elorza MDLA, Frutos G. Improvement of gentamicin poly (D, L-lactic-co-glycolic acid) microspheres for treatment of osteomyelitis induced by orthopedic procedures. Biomaterials 2007; 28: 877-885.
- Sousa F, Luzardo-Álvarez A, Pérez-Estévéz A, Seoane-Prado R, Blanco-Méndez J. Development of a novel AMX-loaded PLGA/zein microsphere for root canal disinfection. Biomedical Materials 2010; 5: 055008.
- Imbuluzqueta E, Elizondo E, Gamazo C, Moreno-Calvo E, Veciana J, Ventosa N, Blanco-Prieto MJ.Novel bioactive hydrophobic gentamicin carriers for the treatment of intracellular bacterial infections. Actabiomaterialia 2011; 7: 1599-1608.
- Prior S, Gamazo C, Irache J, Merkle H, Gander B. Gentamicin encapsulation in PLA/PLGA microspheres in view of treating Brucella infections. International Journal of Pharmaceutics 2000; 196: 115-125.
- Blanco-Prieto M, Lecaroz C, Renedo MJ, Kunkova J, Gamazo C. In vitro evaluation of gentamicin released from microparticles. International journal of pharmaceutics 2002; 242: 203-206.
- Habraken W, Liao H, Zhang Z, Wolke J, Grijpma D, Mikos A, Feijen J, Jansen J. In vivo degradation of calcium phosphate cement incorporated into biodegradable microspheres. Actabiomaterialia 2010; 6: 2200-2211.
|